2021 1.0.1 Enables Collaborative BIM and CAD Workflows, New Functionalities for Metal 3D Printing and Sheet-Metal Manufacturing, and More
Release Highlights:
- Unite Architectural Form and Engineering Function: Spatial Offers One-Stop Components for Collaborative BIM Workflows
- Accelerate 3D Printing: Automated Lightweighting and Support-Creation with CGM Modeler
- Fast Sheet-Metal Manufacturing: Quickly Detect Bends and Unfold Parts with CGM Modeler
- Find Your Way Around Tessellated Geometry: Spatial Now Supports Canonic Edge and Face Recognition in Polyhedral Models
- Enable CAD Workflows in Parallel: Quickly Reconcile Multiple Design Changes to the Same Model with Entity Comparison in the 3D ACIS Modeler
- Let the 3D ACIS Modeler Quickly Simplify Your CAD: New Multi-Threaded and Canonical-to-Canonical Simplifications at Your Service
- Other Highlights in 2021 1.0.1
Unite Architectural Form and Engineering Function:
Spatial Offers One-Stop Components for Collaborative BIM Workflows
On-going advances in software permit increasing integration of architectural and engineering workflows to extend the traditional functionality of BIM applications. As a result, architects and engineers can more easily collaborate virtually to optimize architectural form and engineering function — while changes are still easy and before any expensive procurement and construction begin.
Consider the layout of a heating, ventilation, and air-conditioning (HVAC) system for a building. Initial designs for complicated systems like HVAC sometimes do not function as originally intended – especially if architectural changes to the building are made. To solve this issue, one can conduct virtual “trial and error” tests of the performance of HVAC designs. In doing so, engineers can flexibly test designs early and thus minimize project risk.
1. Import and Filter IFC Building Model with 3D InterOp
![]() |
Import and filter IFC model of building |
Spatial's 3D InterOp suite of translators enable a key first step in the collaboration between architects and engineers by importing a variety of BIM formats. These formats range from standards like IFC to commercial formats such as DXF/DWG and packages like Revit. For example, BIM applications can quickly import complex IFC models of office buildings with many stories, each story containing various structural elements (walls, columns, floors, doors, windows) as well as mechanical and electrical systems.
![]() |
Filter specific story of IFC file to only show structural elements like walls, windows, and doors |
3D InterOp now enables the activation of filters to only import specific information contained in an IFC file. For example, applications can filter IFC models for specific stories and structural elements as well as associated data for electrical, water, fire-suppression and heating, ventilation, air-conditioning (HVAC) systems. Applications enabled by 3D InterOp can directly access only those specific subsets of data needed in an IFC file, which are relevant for downstream workflows.
With the option of upstream filtering in 3D InterOp, architects and engineers can more efficiently leverage specific information in complex models. Such flexibility greatly enhances applications' productivity – especially for large projects with many levels of embedded data.
2: Design HVAC System with CGM Modeler
Assume that a specific floor of the office building does not yet have an HVAC system. To design an HVAC system for a specific floor, an engineer must add relevant components like ducts, vents, blowers, pipes, pumps, and heat-exchangers to the architectural model.
Both 3D InterOp and CGM Modeler can be employed by a BIM application to quickly design an HVAC system. For example, 3D InterOp allows the import of models for blowers, valves, pumps, compressors, and heat-exchanges in a variety of standard CAD formats such as IGS, STP, and JT as well as commercial packages like SolidWorks, CATIA, NX, Inventor, and Creo. In addition, CGM Modeler enables the creation of commands to automate the task of creating repetitive HVAC components such as ducts, vents, and pipes into the architectural model. As a result, engineers can quickly integrate HVAC components into one design in an existing architectural model.
Finished HVAC design for 3rd floor
3. Virtual Testing: Create Mesh with 3D Precise Mesh
Consider a hot summer day in the afternoon. Will the newly-designed HVAC system cool rooms to comfortable temperatures for employees while also conserving energy costs? To answer questions such as this, the engineer can conduct a computational fluid-dynamics (CFD) analysis to determine the temperatures and airflow in rooms with the HVAC system turned on during a hot summer day.
The first step in conducting such a CFD analysis within a BIM application requires meshing of rooms, enabled by 3D Precise Mesh from Spatial. The meshes can include not only structural elements like walls, windows, and doors but also specific components like ducts, vents, pipes, and radiators for HVAC systems, and even furniture and office equipment for more detailed analyses.
![]() |
Volume Mesh of Rooms for CFD Analysis |
The process of creating volume meshes from room geometry for a CFD analysis can be a challenge – especially if details such as components and furniture are considered. However, 3D Precise Mesh enables robust and high-quality meshing capabilities that make this task easier.
For example, 3D Precise Mesh automatically creates surface meshes that respect the internal surfaces of rooms depending on detail: simpler for just structural elements to more complex for components, office equipment, and furniture. From the surface meshes, 3D Precise Mesh then creates volume meshes of the rooms’ interiors. This two-step process is more robust than simply creating a volume mesh.
Furthermore, 3D Precise Mesh enables associativity between the meshes and underlying geometry. The application can automatically incorporate any design changes made to the architectural model or the HVAC system into the meshes. As a result, architects and engineers can quickly iterate design changes to geometry without the need to manually update meshes each time.
The meshing process can be guided by various options embedded in 3D Precise Mesh that allow applications to quickly clean geometry and ensure that the resulting volume meshes are watertight. In doing so, 3D Precise Mesh enables the quick creation of quality meshes with as much geometric detail as necessary.
In addition, meshes created with 3D Precise Mesh can be fine-tuned to account for specific cases such as high-speed flows. High-speed flows in rooms might occur where duct outlets from the HVAC system are present. In such cases, 3D Precise Mesh now enables the automatic creation of tunable boundary-layer meshes to obtain more accurate results.
4. Virtual Testing: Prepare Mesh
Once the volume meshes of rooms have been created, the engineer adds boundary conditions to the meshes. The boundary conditions can be in the form of internal wall temperatures, inflow/outflow rates for vents and water lines, airflows through open doors and windows or leakage around closed doors and windows, solar loading from windows, and any internal heat-sources such as employees or office equipment like computers.
5. Virtual Testing: Run Solver and Analyze Results
Now, the engineer is ready to virtually determine if the initial design of the HVAC system can efficiently handle a hot day. The engineer directs a CFD solver embedded in the BIM application to calculate temperatures and airflows within the rooms at different times. The engineer can analyze the results to determine if any modifications to the HVAC system or rooms’ architectural configurations are necessary.
Thanks to associativity between the meshes and underlying geometry, with any design changes to the architectural model or HVAC system made, the meshes can be automatically updated, and a new simulation conducted quickly. After one or more such design iterations, an optimal layout for an HVAC system can be achieved before the expensive construction begins and any design changes become increasingly difficult.
Spatial’s suite of 3D InterOp translators and 3D Precise Mesh meshing components enable multi-role teams of architects, interior designers, supply-chain purchasers, engineers, and technicians to collaboratively work together and conduct “trial and error” tests of designs.

Click to learn more about 3D InterOp, CGM Modeler, and 3D Precise Mesh.
Accelerate 3D Printing:
Automated Lightweighting and Support-Creation with CGM Modeler
Digital design allows engineers to optimize virtual parts not only for in-service performance — weight, reliability, appearance — but also for manufacturability and ultimately cost. Parts intended for 3D printing offer new design opportunities previously unobtainable by traditional subtractive manufacturing methods and are particularly ideal for virtual optimization.
Spatial’s CGM Modeler allows software applications for 3D printing to automate the virtual optimization of parts for both service and manufacturability. In particular, Spatial enables not only the “lightweighting” of parts but also the creation of supports during printing.
With functionality embedded in CGM Modeler, developers can quickly write user-friendly 3D printing applications that quickly eliminate unnecessary material yet still maintain necessary stiffness by hollowing solid volumes and adding stress-bearing patterns such as ribs, buttresses, and even complex lattices.
1. Import Part: Normal Quick-Release Lever for Bicycle Wheel
![]() |
Normal quick-release bicycle lever for commuter bicycle |
Consider the example of the CAD model of a quick-release lever for the wheel of a normal commuter bicycle, which was imported by 3D InterOp. The lever is solid because weight is not really important. The important attributes for this part are cost, ease-of-use, and robustness. As a result, the lever is simply designed and can be die-cast from solid steel or aluminum blanks and then machined.
2. Optimize Part by Lightweighting: Create Internal Honeycomb Shell
![]() |
Super optimized “lightweighted” bicycle lever with a shelled honeycomb lattice |
However, consider a fancy high-end touring bicycle and a very picky professional racing team as a customer. For such a racing team, weight is paramount. Hence, the quick-release lever must be as light as possible but also functional and certainly robust. What to do?
3D metal printing provides the answer. 3D printing allows the manufacture of lightweight, stiff parts, which are impossible to make with traditional manufacturing methods. For a quick-release lever for professional racers, the original lever can be optimized by creating a shell structure with an internal honeycomb lattice to eliminate weight while preserving stiffness and ultimately robustness.
With the aid of CGM Modeler’s various modeling operators, a honeycomb structure is quickly created and trimmed with the external surfaces of the original lever. Then the original lever is shelled to the desired thickness and subsequently united with the trimmed honeycomb structure. Now we have a lightweight yet stiff lever custom-designed for a professional racing team.
3. Orient Part for 3D Printing
![]() |
Orient lightweighted lever with CGM Modeler for 3D printing (oriented lever highlighted) |
After the lever is optimized by lightweighting, it can be oriented on a virtual tray for 3D printing. CGM Modeler enables an application to select an appropriate orientation for the user depending on various user-controlled geometric options to minimize support material.
4. Create Perforation Patterns and Supports and Anchors for 3D Printing
![]() |
Supports created by the CGM Modeler for the lightweighted lever |
Now, users of the 3D printing application are ready to start the CGM Modeler-enabled process of creating supports to hold the lightweighted lever during printing. The supports not only secure the part but also ensure adequate heat-transfer to minimize thermal warping while ensuring easy detachment and deburring after printing. Supports, both simple and angled, with a variety of perforation patterns and attachment methodologies, are easily created with the aid of CGM Modeler’s new support operators.
![]() |
Supports created by the CGM Modeler for the lightweighted lever |
First patterns for supports and anchors are created with CGM Modeler. In this example, only one pattern is created for all supports and anchors. However, numerous support patterns can be created depending on individual supports and anchors to aid in heat-transfer and detachment. Secondly, several attachment locations on the bicycle lever are defined by CGM Modeler. Thirdly, CGM Modeler creates a series of simple and knee supports and anchors between the bicycle lever and a holding tray beneath the part. These supports and anchors will hold the part during printing while conducting heat away from the part to prevent any warping.
5-6: Print and Finish Part
Now the optimized lever is ready for printing with stiffness maintained and adequate supports and anchors defined. After printing, the supports can be easily removed, and the lever finished (e.g., deburred). It is then ready for use. We wish your racing-team customer much success with the new high-performance, lightweighted, quick-release lever!
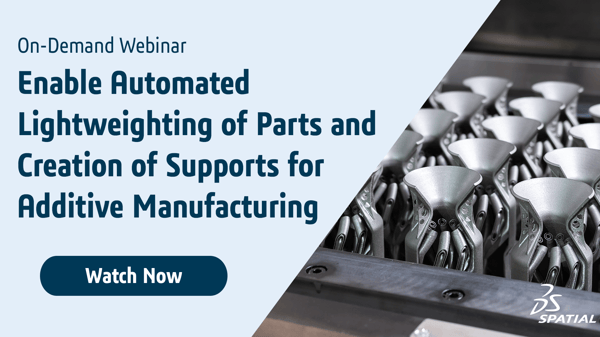
Click for more information on CGM Modeler.
Fast Sheet-Metal Manufacturing:
Quickly Detect Bends and Unfold Parts with CGM Modeler from Spatial
Sheet-metal is often the obvious choice for a myriad of industrial uses, ranging from brackets and enclosures to ducting and car bodies. An important element of sheet-metal manufacturing is the ability to virtually reverse the design process to unfold CAD parts and lay the original sheet-metal flat. Features can then be removed, and the exact size of the sheet-metal blank can be determined and optimally nested within standard sheets for automatic cutting.
The entire workflow for sheet-metal parts, from the detection of bends and virtual unbending to defeaturing, can be implemented into any CAD-CAM software-application with Spatial’s powerful CGM Modeler.
![]() |
Desktop computer housing manufactured from sheet-metal |
1. Import Part: Sheet-Metal Housing for Desktop Computer
Consider for example, the sheet-metal housing of a desktop computer. The housing contains various holes and other cutouts for electrical connections and cooling.
2. Automatically Detect Bends
![]() |
Cylindrical bends of computer housing detected by CGM Modeler |
CGM Modeler begins the process of unbending and defeaturing the housing by automatically detecting cylindrical bends in the model. Various options control the bend-detection process to provide the application utmost flexibility. After the bends are detected, bend objects are created, and the application can interrogate each bend for geometric properties, including type (cylindrical or conical), radii (minimum and maximum), and bend angles.
![]() |
Unbending mid-process: several bends unfolded |
3. Unfold Bends
After CGM Modeler detects the bends, the housing is ready to be unfolded. Additional functionality in CGM Modeler in the form of an unbend operator sequentially unfolds each detected bend. Several optional parameters in the operator control the unbend process.
![]() |
Unbending completed process: all bends unfolded |
![]() |
Feature removal mid-process: holes removed |
4. Remove Holes and Cut-Outs
After the computer housing has been unfolded, CGM Modeler removes holes and other cut-outs to obtain the original sheet-metal blank. The process of removing such features follows a similar process to unbending. First, the holes and cut-outs are detected by feature-detection functionality embedded in CGM Modeler. Then the holes and cut-outs are removed sequentially, leaving just the external perimeter of the original unfolded housing.
![]() |
Feature removal completed process: all features removed (holes and cut-outs) |
![]() |
Sheet-metal blank overlayed on unfolded/defeatured computer housing |
5. Determine the Size of the Sheet-Metal Blank
After all the holes and cut-outs have been removed, CGM Modeler can be used to determine the size of the sheet-metal blank required to manufacture the computer housing. A bounding box is created from the external perimeter of the unfolded and defeatured housing. This bounding box determines the minimum size of the sheet-metal blank. After the blank's size is determined, the application can orient and nest this and other unfolded/defeatured designs onto standard sheet-metal rolls for automated cutting.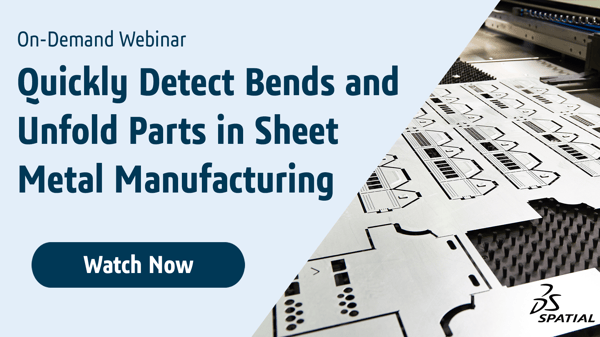